- Condenser Design Calculation Excel Spreadsheet
- Condenser Design Excel
- Condenser Design Calculation Excel Formula
- Condenser Design Calculation Excel Template
- Condenser Calculation
- Condenser Design Calculation
For outlet temperature calculations. Nonetheless, neither ε-Ntu or LMTD approaches can address the heat transfer / pumping power tradeoffs, which is the crux of a balanced heat exchanger design. For this purpose, Bejan (1987) established the thermodynamic design.
Condenser Calculation, Using Thermo Utilities, MS Excel Add-ins
A surface condenser is required to deal with 15 000 kg/h wet steam. Wet steamtemperature is 37 C and enters the condenser with 0.95 quality (dryness fractionis 0.95). The water used for cooling has the following data:
- Varying the Air velocity (m/s) by condenser fan. At differentAir velocity different temperature are calculate like temperature at compressor inlet and outlet,temperature after condenser and also temperature after evaporator. Fig.5 Experimental setup 6. Result and discussion From above study of design optimization of condenser.
- Design of condenser 1. Introduction. A condenser is a type of heat exchanger in which vapors are transferred into liquid state by removing the latent heat with the help of a coolant such as water. Condensers may be classified into two main types: 1.
- Inlet pressure 1.20 bar, inlet temperature 7 C
- Outlet pressure 1.013 bar, outlet temperature 22 C
Calculate the flow rate of cooling water and tube surface of the condenser.Assume that the cooling water velocity through tubes is 1.5 m/sInputs | Units | |
Wet steam inlet temperature | 37 | C |
Steam quality | 0,95 | . |
Cooling water inlet temperature | 7 | C |
Cooling water inlet pressure | 1,2 | bar |
Cooling water outlet temperature | 22 | C |
Cooling water outlet pressure | 1,013 | bar |
Steam mass flow | 15000 | kg/h |
Cooling water speed | 1,5 | m/s |
Outputs | . | . |
Wet steam pressure | 0,06274 | bar |
Enthalpy of steam at inlet | 2448,28 | kJ/kg |
Entropy of condense | 154,92 | kJ/kg |
Rejected heat/hour | 34400441,72 | kJ/h |
Rejected heat/second | 9555,68 | kJ/s = kW |
Enthalpy of water at inlet | 29,53 | kJ/kg |
Enthalpy of water at outlet | 92,31 | kJ/kg |
Water mass flow | 547885,14 | kg/h |
The overall heat transfer coefficient can be calculated according to BEAMAor HEI standards.
BEAMA: British Electrical and Allied Manufacturers Association
Condenser Design Calculation Excel Spreadsheet
BEAMA publication on the recommended practice for design of surface typesteam condenser
HEI: Heat Exchanger Institute
HEI standards for steam surface condensers
Outputs | Unit | |
Heat transfer coeff.(BEAMA) | 2,95235 | KW/m2,K |
Logarithmic mean temp. diff. | 49,83 | degree K |
Required area | 64,96 | m2 |
Outputs | Unit | |
Heat transfer coeff.(HEI) | 3,60991 | kW/m2,K |
Required area | 53,12 | m2 |
Tubular condensers used in power plant to condense exhaust steam are known as Surface Condensers. However, there are many other applications in which condensers are used, and a wide variety of condenser types has been developed.
The direct-contact condenser is one in which the coolant is brought into contact with the vapor. It has the advantage of low cost and simplicity of mechanical design, but its use is restricted to those applications in which mixing of the vapor and coolant is permissible.
The various types of direct contact condensers are:
The Spray Condenser. The coolant is sprayed, using nozzles, into a vessel to which the vapor is supplied. This is shown schematically in Figure 1. It is important that the spray nozzles and vessel are designed to produce a fine spray of liquid (to give a large interfacial area for heat transfer), and a long enough residence time of liquid droplets in the vessel.
The Baffled Column. This is similar to the spray condenser, except that the coolant is directed to flow over a series of trays in a column (see Figure 2). The vapor is supplied to the bottom of the column. It has the advantage of countercurrent flow of vapor and coolant, though care must be taken to avoid flooding. (Flooding is an unstable condition when the vapor flow is such that the downward flow of condensate is interrupted and held up.)
The Packed Column. A packed column may consist of tightly-packed metal rings to increase the interfacial area for heat transfer. Liquid is supplied to the top of the column and vapor is supplied to the bottom. The disadvantage of this type of condenser is that the pressure drop is higher than in other types of direct-contact condenser.
The Jet Condenser. This is a device in which a jet of liquid is directed into a vapor stream, usually with the objective of desuperheating the vapor. A jet of liquid is injected into a pipeline carrying vapor via a small bore pipe and a nozzle located at the center line. The liquid is usually injected in counterflow to the vapor.
The Sparge Pipe. The sparge pipe consists of a pipe with holes for injecting bubbles of vapor into a pool of liquid. This is a simple method of condensing a vapor, but there are practical problems associated with generating a good distribution of bubbles of small size, which are required for efficient heat transfer.
Figure 1. Spray condense.
Figure 2. Tray type condenser. Source: G. P. Hewitt, G. L. Shires, and T. Bott Process Heat Transfer (1994).
The design of direct-contact condensers is well described by Pair (1972). Most equipment of this type is designed on the basis of empirical information from experimental and operating data.
Shell-and-tube condensers are used extensively in the process industries, typically to condense the overhead vapor from a distillation column. There are three main types.
The crossflow condenser is similar to the surface condenser. It consists of a shell containing tubes through which the coolant flows. The shell-side flow path is designed such that the vapor flows mainly in crossflow direction to the tubes. The crossflow condenser is typically used for low-pressure applications, in which there is a large volume flow of vapor and a low-pressure drop is required.The tubes are supported at intervals by plates to prevent sagging of the tubes and to avoid vibration.The vapor enters at the top of the shell. Often, more than one nozzle is used to minimise pressure loss and promote good distribution.It is particularly important to ensure that the crossflow condenser is properly vented.
The baffled shell-and-tube heat exchanger, with shell-side condensation, is the most common type of condenser used in the process industries. It is most often mounted horizontally. A typical shell-side condenser is shown in Figure 3. This is a shell of the TEMA E-type in which the vapor enters at one end of the shell and flows to the outlet end, where the condensate and any uncondensed vapor and non-condensing gases are removed.
Figure 3. Horizontal shell-side condenser. Source: G. F. Hewitt, G. L. Shires, and T. Bott Process Heat Transfer (1994).
The baffles are normally plates with a single segmental cut. The cut is usually vertical to allow the condensate to flow along the bottom of the shell to the outlet. Double segmental baffles may be specified to achieve reduced pressure drop. The space between the baffles is determined by considerations such as:
The tubes must be supported to avoid tube vibration;
Pressure drop depends on vapor velocity, and hence on baffle spacing;
The resistance to heat transfer due to the presence of noncondensing gases is inversely proportional to the vapor velocity.
High vapor velocities can reduce resistance to heat transfer through the shear effect on the condensate film.
Thus, if adequate pressure drop is available, it may be possible to reduce baffle spacing to obtain increased heat transfer rates.
The size of the baffle cut is generally chosen such that the vapor velocity in the baffle window is roughly equal to that in the overlap zone between the baffle plates.
The number of tube passes is determined by the required coolant velocity. Plain tubes are generally used, though low-finned tubes can be used to obtain increased heat transfer rates when the condensate resistance to heat transfer with plain tubes is significantly greater than that of the coolant.
The vapor vent should be situated at the end of the vapor flow path. A typical vent location is shown in Figure 3.
It is possible to use other TEMA shell types, such as the J-type, to minimize pressure drop. A possible configuration for low pressure drop is the J-shell with double segmental baffles.
Condensation on the tube-side is preferred when the coolant is a gas, such as air. It may also be preferred if the condensing fluid is at a higher pressure than the coolant, since it is usually less expensive to contain a higher pressure inside tubes than inside a shell. An air-cooled condenser is typical of a tube-side condenser. It consists of a tube bundle, normally with finned tubes, over which air flows in crossflow. The air flow is driven by fans, either in forced- or induced-draft mode. A typical forced-draft, air-cooled condenser is depicted in Figure 4.
Figure 4. Schematic of air-cooled condenser operating in forced-draft mode. Source: G. F. Hewitt, G. L. Shires, and T. Bott Process Heat Transfer (1994).
Condenser Design Excel
If the tubes are vertical, the two configurations in common use are:
Cocurrent downward vapor and liquid flow;
Countercurrent flow with the vapor flowing upward and the liquid flowing downward. This is commonly known as the reflux condenser.
It is not advisable to attempt cocurrent upward vapor and liquid flow unless there is a high vapor velocity at the outlet of the tubes.
The reflux condenser must be designed such that vapor velocity is less than the flooding velocity. This is a limiting velocity above which drainage of condensate is irregular. (See Flooding and Flow Reversal.)
Area calculation.
In condensation of a single pure vapor, provided that the pressure drop is small compared to the absolute pressure, the temperature of the condensing stream is a constant value determined by the saturation pressure.
If the coolant is single-phase, and if the overall heat transfer coefficient is reasonably constant, then the assumptions underlying the “logarithmic mean temperature difference (LMTD)” are valid. (See Mean Temperature Difference.) This means that the surface area requirement, A, of the condenser can be determined from:
where QT is the total heat load and U is the mean overall heat transfer coefficient.
In condensation from mixtures, with or without a noncondensing gas, the variation of the equilibrium temperature with enthalpy can be highly nonlinear. Also, the heat transfer coefficient of the condensing stream can vary by an order of magnitude over the condensing path. This means that it is not possible to assign a single representative temperature difference and overall heat transfer coefficient to the exchanger, and that a zonal or stepwise calculation of the surface area is required.
The thermal design of condenser is therefore considerably more complicated than that of a single-phase heat exchanger.
Figure 5 shows a typical temperature/enthalpy relationship for a mixture which is superheated at entry. This relationship, and the corresponding physical properties, are normally obtained from specialist computer programs which perform vapor-liquid equilibrium calculations and determine the compositions of the vapor and liquid phases along the condensing path. The corresponding relationship for a single-phase coolant flowing in a single pass in counterflow to the condensing stream is also shown.
Condenser Design Calculation Excel Formula

Figure 5. Example of temperature/enthalpy curves for a condensing stream and coolant, showing subdivision into zones.
Condenser Design Calculation Excel Template
An outline of the procedure to determine the surface area requirement for such a condensing duty is as follows:
Divide the temperature/enthalpy diagram into a number of zones such that the curves of both the condensing stream and the coolant can be regarded as being reasonably linear. An example of how this is done is shown in Figure 5.
Specify the principal geometric parameters of the design, such as the number of tubes, tube outside diameter and wall thickness, tube pitch, baffle cut, baffle pitch and shell diameter.
Calculate the local overall heat transfer coefficients at the zone boundaries.
For each zone, calculate the zonal overall heat transfer coefficient, Uz, from the arithmetic average of the local overall coefficients at the zone boundaries.
Calculate the LMTD for each zone from the temperatures at the zone boundaries.
Calculate the surface area requirement for each zone by applying Eq. (1) above.
Calculate the total surface requirement by summing the zonal surface areas.
The design process consists of repeating the above process, varying the main geometrical parameters to both meet the pressure drop constraints and minimize either area or cost. The procedure is generally carried out using a computer program.
If, as is often the case, condensation occurs on the shell-side with multiple tube-side passes, the calculation of temperature profiles and zonal surface areas is more complicated. A suitable methodology is described by Bell and Ghaly (1972).
The above procedure for calculating surface area requires evaluation of the local heat transfer coefficient at the zone boundaries. This, in turn, requires calculation of:
The coolant heat transfer coefficient;
The resistance to heat transfer due to the tube wall;
The condensate heat transfer coefficient associated with heat transfer through a film of condensate on the tube wall;
The resistance to heat transfer associated with the presence of non-condensing gases, or with a mixture of more than one vapor.
Condenser Calculation
In addition, it is necessary to estimate the resistance to heat transfer due to fouling on both sides of the tube wall. (See Fouling and Fouling Factors.)
Calculation of the condensate heat transfer coefficient depends strongly on condenser geometry. Appropriate methods are described by Hewitt, Shires and Bott (1994), who also prescribed the calculation of the gas-phase resistance. Use of a computer program is required for all but the simplest calculations.
The pressure gradient in condensers is due to both frictional and accelerational effects. The frictional effect, which gives rise to a pressure loss, can be calculated by applying a two-phase multiplier to the pressure gradient for single-phase flow. The accelerational effect gives rise to a pressure increase due to the deceleration of the flow. It is usually only significant at low pressures, and can be calculated on the basis of the vapor flow rate alone.
Some types of plate heat exchangers, which have been traditionally used in other applications, are increasingly used as condensers. For example, the plate-and-frame and brazed-plate heat exchangers, have advantages of lower cost and lower fluid inventory. The use of plate heat exchangers as condensers is discussed by Kumar (1983). Normally, a special plate passage and inlet port configuration will be required to handle the high-vapor volume flow rate at a condenser inlet.
The plate-fin heat exchanger, traditionally used in cryogenic applications, can also be applied to some general condensing applications in which compactness is required.
Removal of gases
It is important to provide a condenser with a vent for removal of gases, either during start-up and/or for continuous operation. The vent must be located near the end of the vapor flow path. It is particularly important in crossflow shell-and-tube condensers to avoid regions of very low vapor velocity, where stagnant zones of noncondensing gas can form and consequently render some of the heat exchanger surface ineffective.
The condensate drain from a condenser must be carefully designed to ensure that it is adequately-sized for the condensate flow rate, and to avoid entrainment of uncondensed vapor or gas into the condensate pipework.
Condenser Design Calculation
Fogging in a condenser is due to the formation of tiny droplets of liquid in the vapor. Fog formation can occur when the temperature of the vapor-gas mixture falls significantly below the local saturation temperature during the condensation process. This tends to happen when the transport properties of a vapor-gas mixture and the process conditions are such that more heat than mass is removed from the mixture. Fog formation represents undesirable loss of product and may, in some circumstances, represent a pollution problem. The actual onset of fog formation will depend on the presence or otherwise of nucleation sites. Removal of fog may require special separation methods. Steinmeyer (1972) gives a good practical account of how fog forms and how its effects can be minimized.
REFERENCES
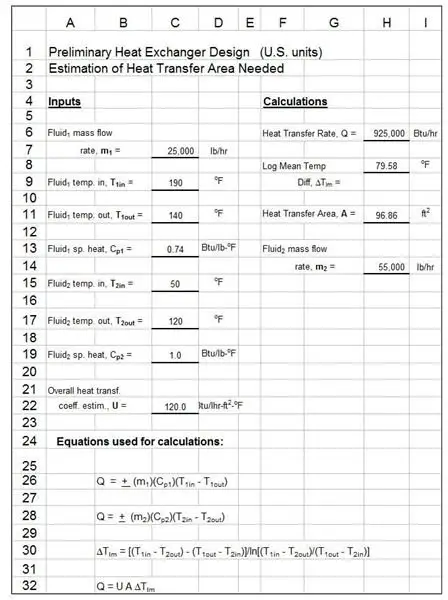
Bell, K. J. and Ghaly, M. A. (1972) An Approximate Generalised Design Method for Multicomponent/Partial Condensers, AIChE Symp. Series, 131 (69), 72.
Fair, J. R. (1972) Designing Direct-Contact Coolers/Condensers, Chem. Engng., 12 June, 91-100.
Hewitt, G. F., Shires, G. L., and Bott, T. R. (1994) Process Heat Transfer, CRC Press, Boca Raton, Florida.
Kumar, H. (1983) Condensation Duties in Plate Heal Exchangers, Instn. Chem Engnrs Symposium Series, 75, 2, 1275.
Steinmeyer, D. E. (1972) Fog Formation in Partial Condensers, Chem. Engng Progress, 68, 7, 64-68.
References
- Bell, K. J. and Ghaly, M. A. (1972) An Approximate Generalised Design Method for Multicomponent/Partial Condensers, AIChE Symp. Series, 131 (69), 72.
- Fair, J. R. (1972) Designing Direct-Contact Coolers/Condensers, Chem. Engng., 12 June, 91-100.
- Hewitt, G. F., Shires, G. L., and Bott, T. R. (1994) Process Heat Transfer, CRC Press, Boca Raton, Florida.
- Kumar, H. (1983) Condensation Duties in Plate Heal Exchangers, Instn. Chem Engnrs Symposium Series, 75, 2, 1275.
- Steinmeyer, D. E. (1972) Fog Formation in Partial Condensers, Chem. Engng Progress, 68, 7, 64-68.